There is an ongoing drive to create synthetic rubber that can give mechanical performance matching the properties of natural rubber (NR) – excellent strength, tear resistance, and fatigue crack growth resistance – which are attributed to the ability of NR to strain crystallize. I have attended several technical conferences in the tire and rubber field where I have witnessed presentations about new grades of polybutadiene (butadiene rubber (BR)) with high cis-1,4 structure, wherein a claim is made about the improved ability of the BR to undergo strain-induced crystallization (SIC) for better mechanical properties in tires and other rubber applications. Similar statements can be found in technical marketing materials and in patents.1,2 To what extent is this true? This post takes a closer look at this topic.
It is first useful to show why strain-induced crystallization is key to the unique mechanical properties of natural rubber. One of the most conclusive studies on the function of strain-induced crystals to slow the growth of cracks in cyclic fatigue is the work by Brüning et al.3 The movie below from this research shows an edge crack region in NR reinforced with 40 phr of N234 carbon black that is experiencing cyclic deformation from 0% to 70% planar tension at a frequency of 1 Hz. Each pixel at each time in the video represents a wide-angle X-ray diffraction pattern collected in real time while stretching in a synchrotron X-ray beam, with red color used to indicate crystallinity and black for purely amorphous regions. You can see the crystals form during stretching which self-reinforces the rubber at the crack tip region to resist further growth of the crack. These experiments are very revealing, but they are extremely difficult and expensive to perform, as they require scattering analysis expertise, specially designed stretching devices with precise spatial control, and access to one of the few national laboratory sites where synchrotron X-ray studies can be performed.
Stereoregular polymers can crystallize in a window bounded by the glass transition temperature (Tg) and the melting temperature (Tm). When stretched, a polymer can crystallize at temperatures above its normal melting temperature due to melting temperature elevation caused by the well-known chain orientation/entropy effect.4 Chain orientation results in an increase in melting temperature from Tm to Tm,SIC. This is shown schematically in the figure below for NR and BR. The Tg and Tm for BR are significantly lower than the values for NR. Therefore, even after perfecting the structure of polybutadiene to achieve >98% cis-1,4 structure though catalyst and process innovations, BR still has an intrinsic disadvantage compared to NR when it comes to SIC in the common temperature range where durable rubber components are employed.
Gent and Zhang5 recognized the lower Tm,SIC for BR compared to NR for samples crystallized in uniaxially strained conditions. Ultra high cis BR with 98% cis content did not show any evidence of strain-induced crystallization at 23 °C whereas NR clearly exhibited SIC in a study by Kang and coworkers.6 In an investigation by Toki et al.,7 it was necessary to reduce the temperature to 0°C before high cis BR showed a crystalline X-ray scattering pattern at a strain of 5 (500% elongation).
As an aside, I will caution the use of the entropy-driven melting temperature increase as the only explanation for strain-induced crystallization above the normal Tm. Natural rubber is quite a slow crystallizing polymer in the unstretched state, even when annealed in the prime crystallization regime midway between Tg and Tm. In stark contrast, strain-induced crystallization occurs very fast in NR which highlights the complexity of behavior beyond the over-simplification given in the figure above.
So, why is there not more focus on synthetic high cis-1,4-polyisoprene which has the same polymer microstructure and strain crystallization window as NR? The short answer is that the butadiene monomer is much more commercially available than isoprene at many synthetic rubber plants around the world. I remember the early years in my industrial R&D career when I was working with some very talented polymer chemists at Bridgestone / Firestone. I was developing new molecular architectures for improved synthetic rubber to use in various tire compounds. The chemists told me that they could make almost any polymer design I wanted, as long as it could be made from styrene and/or butadiene. This restriction reflected the fact that these two monomers were the commonly available feedstocks at the commercial plants where the polymers would eventually be produced.
In closing, it is a fact that high cis polybutadiene can strain crystallize at sub-ambient temperatures, but it is fiction that it will strain crystallize in the same manner as natural rubber at room temperature and above.
Do you want to see if your rubber will really exhibit strain-induced crystallization in a practical way that is relevant to end-use applications? Contact me (via email: cgrobertson@endurica.com) to learn more about the Non-Relaxing Module in Endurica’s portfolio of testing services that was specifically developed to efficiently highlight SIC effects.8-10
References
1 http://arlanxeo.de/uploads/tx_lxsmatrix/sustainable_mobility_01.pdf
2 S. Luo, K. McKauley, and J. T. Poulton, “Bulk polymerization process for producing polydienes”, U.S. Patent 8,188,201, granted May 29, 2012 to Bridgestone Corporation.
3 K. Brüning, K. Schneider, S. V. Roth, and G. Heinrich, “Strain-induced crystallization around a crack tip in natural rubber under dynamic load”, Polymer 54, 6200 (2013).; movie provided to Endurica LLC by the authors (movie to be used only with permission from Karsten Brüning).
4 P. J. Flory, “Thermodynamics of crystallization in high polymers. I. Crystallization induced by stretching”, Journal of Chemical Physics 15, 397 (1947).
5 A. N. Gent and L.-Q. Zhang, “Strain-induced crystallization and strength of elastomers. I. cis-1,4-polybutadiene”, Journal of Polymer Science Part B: Polymer Physics 39, 811 (2001)
6 M. K. Kang, H.-J. Jeon, H. H. Song, and G. Kwag, Strain-induced crystallization of blends of natural rubber and ultra high cis polybutadiene as studied by synchrotron X-ray diffraction”, Macromolecular Research 24, 31 (2016).
7 S. Toki, I. Sics, B. S. Hsiao, S. Murakami, M. Tosaka, S. Poompradub, S. Kohjiya, and Y. Ikeda, “Structural developments in synthetic rubbers during uniaxial deformation by in situ synchrotron X-ray diffraction”, Journal of Polymer Science Part B: Polymer Physics 42, 956 (2004).
8 K. Barbash and W. V. Mars, “Critical Plane Analysis of Rubber Bushing Durability under Road Loads”, SAE Technical Paper 2016-01-0393, 2016, https://doi.org/10.4271/2016-01-0393.
9 W. V. Mars and A. Fatemi. “A phenomenological model for the effect of R ratio on fatigue of strain crystallizing rubbers”, Rubber Chemistry and Technology 76, 1241 (2003).
10 https://endurica.com/specifying-strain-crystallization-effects-for-fatigue-analysis/
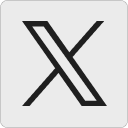

